Chiral phosphoric acid catalyzed redox deracemization of triarylmethanes
Abstract
Described here is the first deracemization of triaryl-substituted carbon stereocenters, which is in contrast to the well-established processes to deracemize monoaryl- and diaryl-substituted ones. This one-pot redox process involves in situ generation of a para-quinone methide intermediate followed by asymmetric reduction by chiral phosphoric acid catalysis. A wide range of highly enantioenriched triarylmethanes could be generated with high efficiency under mild conditions.
Keywords
INTRODUCTION
Deracemization is an attractive strategy to provide access to enantioenriched organic molecules[1-8]. However, direct conversion of the racemic form of a chiral compound to its enantioenriched form is a thermodynamically unfavorable transformation due to the positive Gibbs free energy change as a result of the increased entropy of the reaction system as well as the principle of microscopic reversibility under thermal conditions[9,10]. To overcome this hurdle, various strategies have been devised to achieve successful deracemization[1-8], including the use of excited states (via photochemical condition)[1,2,11-14], reversal of thermodynamics by extrusion of small gas molecules[15,16], and the design of multistep reaction sequence (e.g., kinetic resolution or dynamic kinetic resolution)[17-21]. However, there are limited examples of successful implementation of these strategies, and more efficient methods for this purpose remain in high demand.
Enantioenriched organic molecules with benzylic chirality show broad applications in various fields, including organic synthesis, medicinal chemistry, and materials science[22,23]. In particular, a stereogenic carbon center attached to multiple aryl groups represents an important substructure widely observed in natural products and biologically active molecules[24-28]. In contrast to the well-documented diverse strategies to construct benzylic stereogenic centers, the exploitation of the deracemization approach for this purpose has been underdeveloped in general. Among these limited examples, the majority have dealt with those bearing one aryl group at the benzylic position [Scheme 1a][29-33]. Instead, only very few deracemization protocols have been developed for access to enantioenriched 1,1-diarylalkanes with a diaryl-substituted stereogenic center[34-36]. More disappointingly, to the best of our knowledge, there has been no demonstration of deracemization of triaryl-substituted stereogenic centers, despite the fact that 1,1,1-triarylalkanes are versatile structures in medicinal chemistry. In this context, here we report the first example of this type employing para-quinone methides as the key intermediate.
Scheme 1. Introduction and Reaction Design. p-QMs: para-quinone methides; Ar2 and Ar3: two aryl groups.
Recently, Liu and co-workers have reported a series of elegant organocatalytic redox racemization examples with outstanding performance for the access to enantioenriched chiral molecules bearing benzylic stereogenic centers[34-39]. Inspired by this strategy as well as our previous efforts in the study of asymmetric processes involving para-quinone methides (p-QMs)[40-56], we envisioned that the deracemization of triarylmethane 1 could be potentially achieved by a similar strategy. Specifically, initial oxidation is expected to form the p-QM intermediate. Next, in the same pot, a reductant, as well as a chiral catalyst, would affect the asymmetric reduction of this key intermediate, thereby representing a formal deracemization [Scheme 1d]. The challenges associated with this strategy include not only stereo control which requires discrimination between two aryl groups (Ar2 and Ar3), but also the compatibility of the two steps which involve mutually destructive oxidant and reductant.
EXPERIMENTAL
At room temperature, a solution of the triarylmethane 1 (0.4 mmol) and DDQ (99.0 mg, 0.44 mmol, 1.1 equiv) in CHCl3 (1.44 mL) was charged into an oven dried 4 mL vial. The mixture was stirred for 5 h and then cooled. The catalyst (R)-A3 and the hydrogen source (0.6 mmol, 1.5 equiv) were added to a lower temperature as specified in each case. The mixture was stirred for 96 h. Upon completion, as monitored by TLC, it was concentrated under reduced pressure. The residue was purified by silica gel column chromatography to afford the desired product 2.
RESULTS AND DISCUSSION
The racemic triarylmethane 1a was chosen as the model substrate for the initial study [Figure 1]. The phenol ring serves as the precursor to the p-QM structure. To distinguish the remaining two aryl groups, one of them was substituted with an ortho-methoxy group to provide additional interaction with the catalyst[51-56]. DDQ was used as an oxidant for the first step. Based on TLC analysis, this step could be achieved cleanly in DCE at room temperature within 4 h. Notably, other oxidants, including Ag2O, TEMPO, Mn(acac)3, and O2, could not work as effectively as DDQ. Next, the search for a suitable reductant and a chiral catalyst constituted the key to success. Chiral phosphoric acids were employed as catalysts owing to their well-known performance in such nucleophilic addition reactions[43-66]. Inspired by Akiyama’s pioneering study of using benzothiazoline for CPA-catalyzed asymmetric reduction[57,58], the 2-naphthyl-substituted one H1 was initially used as a reductant[65]. To our delight, this one-pot redox process proceeded smoothly to afford the desired enantioenriched product 2a, essentially in quantitative yields in all the cases. Among all the CPAs evaluated, the BINOL-derived one bearing two 2,4,6-tricyclohexylphenyl substituents at the 3,3’-positions provided the best enantioselectivity (96% ee, entry 3). Other backbones, such as [H8]BINOL and spirocyclic bis(indane)-based SPINOL, did not result in better results (entries 4 and 5). Next, we also compared different hydride sources, including 2-phenyl-substituted benzothiazoline H2, catechol borane H3, and Hantzsch ester H4. Unfortunately, they proved inferior in terms of enantioselectivity (entries 6-8). We next screened other solvents, which indicated that chlorinated solvents are in general good for this reaction. Among them, CHCl3 provided the best enantioselectivity (entry 9). Finally, a lower catalyst loading was also evaluated. With only 0.5 mol% of catalyst A3, the reaction efficiency and enantioselectivity remained excellent (entry 12). Furthermore, a scale-up reaction at a lower temperature (-10 oC) provided the best overall outcome (entry 13).
Figure 1. Optimization of Reaction Conditions[a].
With the optimized conditions [Figure 1, entry 13], we examined the generality of this one-pot deracemization protocol [Figure 2]. Different substituted triarylmethane substrates all participated in this reaction to provide the enantioenriched products with both good yield and excellent enantioselectivity [Scheme 2]. Electron-donating groups and electron-withdrawing groups (e.g., nitro, cyano, halogen, and trifluoromethyl) did not affect the excellent outcome. However, it was found that those electron-poor substrates typically required a higher catalyst loading and/or higher temperature for the reaction to go completion. Thiophene-substituted triarylmethanes [2m and 2o] were also obtained in high enantiomeric excess, demonstrating the compatibility of this mild protocol to heterocycles. In these examples, an ortho-methoxy group was present in one of the aryl rings to provide differentiation between the other arene. It is worth noting that other directing groups, such as fluorine and benzyl ether, could also serve the same purpose[55]. More drastically, discrimination of these two arenes by steric hindrance is also possible. For example, a methyl or ethyl group at the ortho-position also led to good enantioselectivity. Ortho-halogen (Cl or Br) also provided good levels of differentiation. This is noteworthy since these halide groups can be easily converted to many other functionalities. Interestingly, if both ortho-OMe and ortho-F are present in the two arenes, effective discrimination was also observed. Notably, the absolute stereochemistry of product 2f was confirmed by X-ray crystallography.
Figure 2. Reaction Scope[a]. [a] Reaction conditions:1a (0.05 mmol), DDQ (0.55 mmol), H1 (0.075 mmol), catalyst (10 mol%), solvent (1.0 mL). The yield was determined to be > 95% in all the cases by 1H NMR and TLC analyses of the crude reaction mixture; ee value was determined by chiral HPLC analysis. [b] Run with 0.5 mol% of catalyst. Solvent (0.18 mL, c = 0.28 M). [c] Run for 36 h. [d] Run at -10 oC, 1a (0.4 mmol), DDQ (0.44 mmol), H1 (0.6 mmol), solvent (1.44 mL), 96 h.
Scheme 2. Gram-scale Reaction and Product Derivatization. [a] Reaction conditions:1 (0.4 mmol), DDQ (0.44 mmol), H1 (0.6 mmol). Ee was determined by chiral HPLC analysis. Isolated yield. The specified temperature for each example is for the second step (reduction). [b] Run with 0.5 mol% of catalyst. [c] Run with 1 mol% of catalyst. [d] Run with 2 mol% of catalyst. [e] Run with 5 mol% of catalyst.
To further demonstrate the robustness of this process, we carried out a gram-scale reaction of 1a. Under the standard conditions, the desired deracemization product was obtained in 96% yield and 98% ee [Scheme 2]. The ortho-methoxy group in product 2a could also be deprotected to form a free hydroxyl group without erosion in enantiomeric excess. Based on our previous work[65], this bis(phenol) 3a could be further converted to spirocyclic dienone 4 in the presence of PhI(OAc)2 without erosion in its ee value.
CONCLUSIONS
In summary, we have developed the first deracemization approach for efficient access to enantioenriched triarylmethanes, a type of useful structure in medicinal chemistry. In contrast to the well-established deracemization processes for monoaryl- and diaryl-substituted carbon stereogenic centers, limited success has been achieved previously for triaryl-substituted ones. Specifically, herein a redox strategy involving the initial oxidation of racemic triarylmethanes followed by asymmetric reduction has been achieved in a one-pot fashion. With suitable substitution on the arenes, this process proceeds through the key para-quinone methide intermediate. Chiral phosphoric acids have shown excellent capability in catalyzing this process. The reaction features mild conditions and low catalyst loading. This process provided a diverse set of highly enantioenriched triarylmethanes with high efficiency and excellent enantioselectivity. Notably, diverse ortho-substituents on one of the arenes have been demonstrated to be powerful for providing asymmetric discrimination. Further extension of this strategy for more broad applications is expected.
DECLARATIONS
AcknowledgmentsWe thank Dr. Herman H. Y. Sung for his help in structure elucidation by X-ray crystallography.
Authors’ contributionsDesigning the experiments, writing the manuscript, and being responsible for the whole work: Sun JW, Li PF
Performing the experiments and synthesizing the substrates: Liu C, Li ZY
Availability of data and materialsDetailed experimental procedures and spectroscopic data were published as Supplementary Materials in the journal.
Financial support and sponsorshipFinancial support was provided by the National Natural Science Foundation of China (22271242), the Science Technology and Innovation Committee of Shenzhen Municipality (JCYJ20200109141408054), the Hong Kong Research Grants Council (C6012-21G, 16303420, 16309321, 16309722, and 16304322) and Innovation and Technology Commission (ITC-CNERC14SC01) for funding support.
Conflicts of interestAll authors declared that there are no conflicts of interest.
Ethical approval and consent to participateNot applicable.
Consent for publicationNot applicable.
Copyright© The Author(s) 2023.
REFERENCES
1. Shi Q, Ye J. Deracemization enabled by visible-light photocatalysis. Angew Chem Int Ed Engl 2020;59:4998-5001.
3. Aranda C, Oksdath-mansilla G, Bisogno FR, Gonzalo G. Deracemisation processes employing organocatalysis and enzyme catalysis. Adv Synth Catal 2020;362:1233-57.
4. Rachwalski M, Vermue N, Rutjes FP. Recent advances in enzymatic and chemical deracemisation of racemic compounds. Chem Soc Rev 2013;42:9268-82.
5. Voss C, Gruber C, Kroutil W. Deracemisation of secondary alcohols via biocatalytic stereoinversion. Synlett 2010;2010:991-8.
6. Steinreiber J, Faber K, Griengl H. De-racemization of enantiomers versus de-epimerization of diastereomers--classification of dynamic kinetic asymmetric transformations (DYKAT). Chemistry 2008;14:8060-72.
7. Gruber C, Lavandera I, Faber K, Kroutil W. From a racemate to a single enantiomer: deracemization by stereoinversion. Adv Synth Catal 2006;348:1789-805.
8. Pàmies O, Bäckvall JE. Combined metal catalysis and biocatalysis for an efficient deracemization process. Curr Opin Biotechnol 2003;14:407-13.
10. Blackmond DG. "If pigs could fly" chemistry: a tutorial on the principle of microscopic reversibility. Angew Chem Int Ed Engl 2009;48:2648-54.
11. Drucker CS, Toscano VG, Weiss RG. General method for the determination of steric effects during collisional energy transfer. partial photoresolution of penta-2, 3-diene. J Am Chem Soc 1973;95:6482-4.
12. Ouannes C, Beugelmans R, Roussi G. Asymmetric induction during transfer of triplet energy. J Am Chem Soc 1973;95:8472-4.
13. Hölzl-Hobmeier A, Bauer A, Silva AV, Huber SM, Bannwarth C, Bach T. Catalytic deracemization of chiral allenes by sensitized excitation with visible light. Nature 2018;564:240-3.
14. Shin NY, Ryss JM, Zhang X, Miller SJ, Knowles RR. Light-driven deracemization enabled by excited-state electron transfer. Science 2019;366:364-9.
15. Mohr JT, Behenna DC, Harned AM, Stoltz BM. Deracemization of quaternary stereocenters by Pd-catalyzed enantioconvergent decarboxylative allylation of racemic beta-ketoesters. Angew Chem Int Ed Engl 2005;44:6924-7.
17. Nakamura K, Inoue Y, Matsuda T, Ohno A. Microbial deracemization of 1-arylethanol. Tetrahedron Letters 1995;36:6263-6.
18. Voss CV, Gruber CC, Kroutil W. Deracemization of secondary alcohols through a concurrent tandem biocatalytic oxidation and reduction. Angew Chem Int Ed Engl 2008;47:741-5.
19. Liardo E, Ríos-lombardía N, Morís F, González-sabín J, Rebolledo F. A straightforward deracemization of sec -alcohols ccombining organocatalytic oxidation and biocatalytic reduction. Eur J Org Chem 2018;2018:3031-5.
20. Koszelewski D, Pressnitz D, Clay D, Kroutil W. Deracemization of mexiletine biocatalyzed by omega-transaminases. Org Lett 2009;11:4810-2.
21. Meng FJ, Shao BR, Velopolcek MK, Guo X, Feng GS, Shi L. Redox deracemization of phosphonate-substituted dihydropyrimidines. Org Biomol Chem 2021;19:10570-4.
22. Mondal S, Roy D, Panda G. Critical view on the recent enantioselective synthesis of alcohols, amines and related molecules having tertiary benzylic stereocenter. Tetrahedron 2018;74:4619-703.
23. Liu R, Liang R, Jia Y. Construction of benzylic stereogenic carbon centers through enantioselective arylation reactions. Synlett 2018;29:157-68.
24. Hucke O, Gelb MH, Verlinde CL, Buckner FS. The protein farnesyltransferase inhibitor Tipifarnib as a new lead for the development of drugs against Chagas disease. J Med Chem 2005;48:5415-8.
25. Nambo M, Crudden CM. Recent advances in the synthesis of triarylmethanes by transition metal catalysis. ACS Catal 2015;5:4734-42.
26. Mondal S, Roy D, Panda G. Overview on the recent strategies for the enantioselective synthesis of 1, 1-diarylalkanes, triarylmethanes and related molecules containing the diarylmethine stereocenter. ChemCatChem 2018;10:1941-67.
27. Kshatriya R, Jejurkar VP, Saha S. Advances in the catalytic synthesis of triarylmethanes (TRAMs). Eur J Org Chem 2019;2019:3818-41.
28. Mondal S, Panda G. Synthetic methodologies of achiral diarylmethanols, diaryl and triarylmethanes (TRAMs) and medicinal properties of diaryl and triarylmethanes-an overview. RSC Adv 2014;4:28317-58.
29. Huang M, Zhang L, Pan T, Luo S. Deracemization through photochemical E/Z isomerization of enamines. Science 2022;375:869-74.
30. Zhang C, Gao AZ, Nie X, et al. Catalytic α-deracemization of ketones enabled by photoredox deprotonation and enantioselective protonation. J Am Chem Soc 2021;143:13393-400.
31. Zhang Z, Hu X. Visible-light-driven catalytic deracemization of secondary alcohols. Angew Chem Int Ed Engl 2021;60:22833-8.
32. Gu Z, Zhang L, Li H, et al. Deracemization through sequential photoredox-neutral and chiral brønsted acid catalysis. Angew Chem Int Ed Engl 2022;61:e202211241.
33. Chen Q, Zhu Y, Shi X, et al. Light-driven redox deracemization of indolines and tetrahydroquinolines using a photocatalyst coupled with chiral phosphoric acid. Chem Sci 2023;14:1715-23.
34. Chen X, Zhao R, Liu Z, Liu L. Redox deracemization of α-substituted 1, 3-dihydroisobenzofurans. Chin Chem Lett 2021;32:2305-8.
35. Ma Y, Liu X, Mao Y, Huang J, Ma S, Liu L. Redox deracemization of diarylmethyl alkynes. Org Chem Front 2020;7:2526-30.
36. Mao Y, Wang Z, Wang G, et al. Redox deracemization of tertiary stereocenters adjacent to an electron-withdrawing group. ACS Catal 2020;10:7785-91.
37. Chen X, Yan L, Zhang L, et al. Aerobic redox deracemization of α-aryl glycine esters. Tetrahedron Letters 2020;61:152107.
38. Wan M, Sun S, Li Y, Liu L. Organocatalytic redox deracemization of cyclic benzylic ethers enabled by an acetal pool strategy. Angew Chem Int Ed Engl 2017;56:5116-20.
39. Zhang L, Zhu R, Feng A, et al. Redox deracemization of β,γ-alkynyl α-amino esters. Chem Sci 2020;11:4444-9.
40. Li X, Li Z, Sun J. Quinone methides and indole imine methides as intermediates in enantioselective catalysis. Nat Synth 2022;1:426-38.
41. Li W, Xu X, Zhang P, Li P. Recent advances in the catalytic enantioselective reactions of para-quinone methides. Chem Asian J 2018;13:2350-9.
42. Lima CGS, Pauli FP, Costa DCS, et al. para -Quinone methides as acceptors in 1,6-nucleophilic conjugate addition reactions for the synthesis of structurally diverse molecules. Eur J Org Chem 2020;2020:2650-92.
43. Chu WD, Zhang LF, Bao X, et al. Asymmetric catalytic 1,6-conjugate addition/aromatization of para-quinone methides: enantioselective introduction of functionalized diarylmethine stereogenic centers. Angew Chem Int Ed Engl 2013;52:9229-33.
44. Caruana L, Kniep F, Johansen TK, Poulsen PH, Jørgensen KA. A new organocatalytic concept for asymmetric α-alkylation of aldehydes. J Am Chem Soc 2014;136:15929-32.
45. Lou Y, Cao P, Jia T, Zhang Y, Wang M, Liao J. Copper-catalyzed enantioselective 1,6-boration of para-quinone methides and efficient transformation of gem-diarylmethine boronates to triarylmethanes. Angew Chem Int Ed Engl 2015;54:12134-8.
46. Wu H, Wang Q, Zhu J. Catalytic enantioselective pinacol and meinwald rearrangements for the construction of quaternary stereocenters. J Am Chem Soc 2019;141:11372-7.
47. Lin JS, Li TT, Liu JR, et al. Cu/Chiral phosphoric acid-catalyzed asymmetric three-component radical-initiated 1,2-dicarbofunctionalization of alkenes. J Am Chem Soc 2019;141:1074-83.
48. Cheng Y, Fang Z, Jia Y, Lu Z, Li W, Li P. Organocatalytic enantioselective conjugate addition of 2-naphthols to ortho-hydroxyphenyl substituted para-quinone methides: access to unsymmetrical triarylmethanes. RSC Adv 2019;9:24212-7.
49. Zhang L, Han Y, Huang A, Zhang P, Li P, Li W. Organocatalytic remote stereocontrolled 1,8-additions of thiazolones to propargylic aza-p-quinone methides. Org Lett 2019;21:7415-9.
50. Li W, Xu X, Liu Y, Gao H, Cheng Y, Li P. Enantioselective organocatalytic 1,6-addition of azlactones to para-quinone methides: an access to α,α-disubstituted and β, β-diaryl-α-amino acid esters. Org Lett 2018;20:1142-5.
51. Wang Z, Wong YF, Sun J. Catalytic asymmetric 1,6-conjugate addition of para-quinone methides: formation of all-carbon quaternary stereocenters. Angew Chem Int Ed Engl 2015;54:13711-4.
52. Chen M, Sun J. How understanding the role of an additive can lead to an improved synthetic protocol without an additive: organocatalytic synthesis of chiral diarylmethyl alkynes. Angew Chem Int Ed Engl 2017;56:11966-70.
53. Qian D, Wu L, Lin Z, Sun J. Organocatalytic synthesis of chiral tetrasubstituted allenes from racemic propargylic alcohols. Nat Commun 2017;8:567.
54. Ma D, Miao CB, Sun J. Catalytic enantioselective house-meinwald rearrangement: efficient construction of all-carbon quaternary stereocenters. J Am Chem Soc 2019;141:13783-7.
55. Li X, Duan M, Deng Z, et al. Catalytic enantioselective synthesis of chiral tetraarylmethanes. Nat Catal 2020;3:1010-9.
56. Li Z, Li Y, Li X, Wu M, He ML, Sun J. Organocatalytic asymmetric formal oxidative coupling for the construction of all-aryl quaternary stereocenters. Chem Sci 2021;12:11793-8.
57. Zhu C, Saito K, Yamanaka M, Akiyama T. Benzothiazoline: versatile hydrogen donor for organocatalytic transfer hydrogenation. Acc Chem Res 2015;48:388-98.
58. Osakabe H, Saito S, Miyagawa M, Suga T, Uchikura T, Akiyama T. Enantioselective dehydroxyhydrogenation of 3-indolylmethanols by the combined use of benzothiazoline and chiral phosphoric acid: construction of a tertiary carbon center. Org Lett 2020;22:2225-9.
59. Akiyama T, Itoh J, Yokota K, Fuchibe K. Enantioselective mannich-type reaction catalyzed by a chiral brønsted acid. Angew Chem Int Ed Engl 2004;43:1566-8.
60. Uraguchi D, Terada M. Chiral Brønsted acid-catalyzed direct Mannich reactions via electrophilic activation. J Am Chem Soc 2004;126:5356-7.
61. Parmar D, Sugiono E, Raja S, Rueping M. Complete field guide to asymmetric BINOL-phosphate derived Brønsted acid and metal catalysis: history and classification by mode of activation; Brønsted acidity, hydrogen bonding, ion pairing, and metal phosphates. Chem Rev 2014;114:9047-153.
63. James T, van Gemmeren M, List B. Development and applications of disulfonimides in enantioselective organocatalysis. Chem Rev 2015;115:9388-409.
64. Kikuchi J, Terada M. Enantioconvergent substitution reactions of racemic electrophiles by organocatalysis. Chemistry 2021;27:10215-25.
65. Han Z, Zang Y, Liu C, Guo W, Huang H, Sun J. Enantioselective synthesis of triarylmethanes via organocatalytic transfer hydrogenation of para-quinone methides. Chem Commun (Camb) 2022;58:7128-31.
Cite This Article
Export citation file: BibTeX | RIS
OAE Style
Liu C, Li Z, Li P, Sun J. Chiral phosphoric acid catalyzed redox deracemization of triarylmethanes. Chem Synth 2023;3:22. http://dx.doi.org/10.20517/cs.2023.18
AMA Style
Liu C, Li Z, Li P, Sun J. Chiral phosphoric acid catalyzed redox deracemization of triarylmethanes. Chemical Synthesis. 2023; 3(3): 22. http://dx.doi.org/10.20517/cs.2023.18
Chicago/Turabian Style
Liu, Chang, Zhiyang Li, Pengfei Li, Jianwei Sun. 2023. "Chiral phosphoric acid catalyzed redox deracemization of triarylmethanes" Chemical Synthesis. 3, no.3: 22. http://dx.doi.org/10.20517/cs.2023.18
ACS Style
Liu, C.; Li Z.; Li P.; Sun J. Chiral phosphoric acid catalyzed redox deracemization of triarylmethanes. Chem. Synth. 2023, 3, 22. http://dx.doi.org/10.20517/cs.2023.18
About This Article
Special Issue
Copyright
Author Biographies
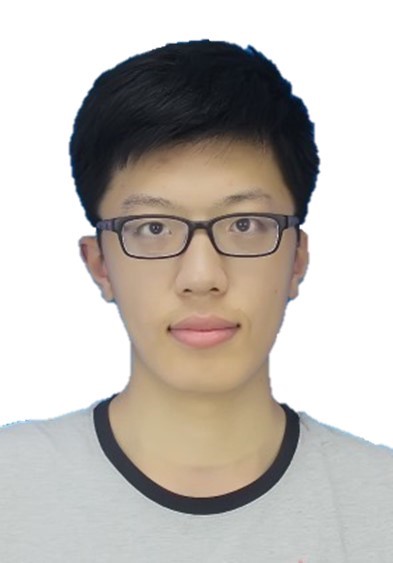
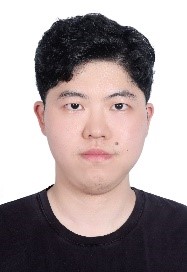
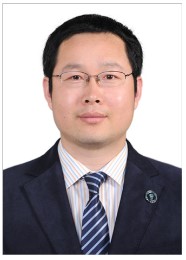
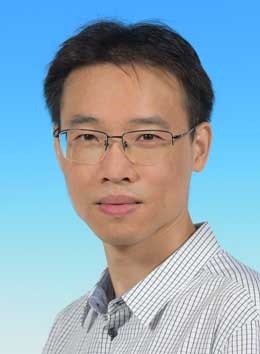
Data & Comments
Data

Comments
Comments must be written in English. Spam, offensive content, impersonation, and private information will not be permitted. If any comment is reported and identified as inappropriate content by OAE staff, the comment will be removed without notice. If you have any queries or need any help, please contact us at support@oaepublish.com.