The recent advances in the contribution of chiral triarylmethanes and tetraarylmethanes with organocatalysts
Abstract
Chiral triarylmethane skeleton is an important structural unit of many known compounds that are widely applied in organic functional materials and pharmaceuticals. Thus, the efficient construction of this class of compounds has attracted intensive attention from chemists. There are two main difficulties in synthesizing this type of compound: (i) the steric resistance of molecular structures would make it hard to be constructed; (ii) there are three similar aryl groups on the stereocenter, which is difficult to achieve stereo-identification. At present, the most common strategy is to introduce the third aryl group into a diarylmethane framework through the asymmetric Friedel-Crafts reaction or addition reaction of electron-rich arenes, so as to construct chiral triarylmethanes. In this review, we summarized the recent developments in the construction of various chiral triarylmethanes and chiral tetraarylmethanes from easily accessible compounds under organocatalytic conditions. This article describes based on the types of electrophilic reagents, mainly including quinone methides, indole imine methides, and azadienes. At the same time, we also emphasize the mechanism of each representative reaction, which might enlighten the future development of this field.
Keywords
INTRODUCTION
Benefiting from the unique structural characteristics, triarylmethanes are widely used in many fields, including pharmaceuticals, functional materials, and organic synthesis[1-4]. In terms of spatial structure, triarylmethane looks like a three-blade propeller. Therefore, the whole triarylmethane molecule is very crowded as there are three bulky aryl groups on the same carbon atom at the same time.
In early studies, the common methods to construct such a challenging molecule were mainly focused on introducing the third aryl group on the methyl position of diaryl methane derivatives through the metal-catalyzed cross-coupling reaction. In 2015, Nambo and Crudden[5] reported a review focused on the synthesis of triarylmethanes using well-defined transition metal catalysts including Pd, Ni, Cu, Rh and Fe. For the above transition metal-catalyzed cross-coupling method, the synthesis of chiral triarylmethanes is purely difficult and relatively less explored, and presynthesized chiral diary methane derivatives are generally used.
Notably, organocatalytic asymmetric synthesis was awarded the 2021 Nobel Prize in Chemistry, which has been demonstrated as one of the most efficient strategies for constructing chiral compounds, benefiting from metal-free, mild condition, excellent stereocontrol, etc. The construction of triarylmethanes and tetraarylmethanes under organocatalytic system has been widely developed in recent years. Among them, the electron-rich aromatic Friedel-Crafts reaction has become an essential tool. In addition to overcoming the potential steric hindrance of molecules, the synthesis of chiral triarylmethanes also requires accurate identification of three similar aryl groups. In other words, the enantioselective transformation can only occur when the three similar aryl groups are clearly divided in a specific chiral environment. Generally, in order to provide the ability to identify similar aryl groups, one of the aryl groups generally undergoes dearomatization to form an active intermediate; after the introduction of the third aryl group, this aryl group is re-formed through aromatization. At present[6], the most widely used intermediates include quinone methides, indole imine methides, azadienes, etc[7-10]. Although Mondal et al. had already reviewed the synthesis of diarylmethanes and triarylmethanes in 2017, including scientific reports of chiral triarylmethanes, many excellent works have emerged in recent years, which are worth summarizing again[11]. In this review, we summarize the synthetic methods for chiral triarylmethanes implemented by organocatalysts, and we discuss the mechanism of each reaction in detail, especially the chirality-determining steps. Generally, reactions need a bifunctional organocatalyst that can grab the active intermediate and nucleophilic substrate into its chiral pocket through the hydrogen bonds, and then undergo the enantioselective functionalization to provide the final chiral triarylmethanes [Figure 1]. Meanwhile, we also summarize a small number of reports on the synthesis of chiral tetraarylmethanes via a similar reaction manner. The potential applications of some products were also highlighted.
CONSTRUCTION OF CHIRAL TRIARYLMETHANES WITH QUINONE METHIDES
Quinone methides (QMs) are very short-lived, polar and highly reactive synthetic intermediates that easily participate in conjugate additions[12-19]. The common structure of QMs consists of a cyclohexadiene ring and an exocyclic methylene group with a reactive carbonyl group binding either on the ortho or para position. Therefore, QMs usually have two topological isomers: one is ortho-quinone methides (o-QMs) and the other is para-quinone methides (p-QMs)[7]. Over the past few decades, the synthetic potential and properties of o- and p-QMs have been widely explored because of their potential applications in bioactive compounds and natural product synthesis. For the conjugate additions of QMs, if electron-rich arenes are employed as nucleophiles, it would provide an efficient route for the asymmetric construction of triarylmethanes, as shown in Scheme 1. Generally, the carbonyl group of QMs can form a hydrogen bond with the organocatalysts, which is crucial in obtaining superior enantioselectivity.
o-QMs. In 2014, Schneider and co-workers[6] disclosed a chiral phosphoric acid (CPA)-catalyzed highly enantioselective 1,4-addition of in situ generated o-QMs with indoles, providing an efficient protocol for the synthesis of broadly substituted chiral triarylmethanes [Scheme 2]. This process has excellent functional group tolerance. A broad set of heteroaryl-substituted triarylmethanes 2b were obtained in good yields and enantioselectivities, irrespective of the electronic properties of the substituents on the indole ring. In the proposed transition state 2-TS-I, CPA-1 played as a bifunctional catalyst which activated both the o-QM and the indole by forming double hydrogen bondings. Then, the indole selectively attacked the o-QM from the re-face because the opposite face was effectively shielded by the neighboring bulky group. In addition, electron-rich 1-naphthols and 2-naphthols were further successfully extended in this strategy replacing the role of indoles. Very recently, Zhang et al. reported enantioselective alkylation of aniline with in situ generated o-QMs catalyzed by chiral phosphoric acid, which further broadened the methodologies of asymmetric synthesis of triphenylmethanes[20].
To generate acyclic all-carbon quaternary stereocenters, tertiary alcohols 3a were selected by Sun et al. as a suitable electrophile to achieve a stereo-control addition with indoles [Scheme 3][21]. This reaction overcame the unfavorable steric hindrance around reactive centers and the competitive elimination process to provide a range of chiral triarylmethanes 3b with excellent yields and enantioselectivities. The author speculated that the in situ generation of o-QMs was the key to forming the all-carbon quaternary stereocenter.
Replacing the oxygen atom of o-QMs with nitrogen, another reactive intermediate, namely aza-ortho-quinone methide (aza-o-QM), can be skillfully obtained. Recently, aza-o-QMs have also been widely used in catalytic asymmetric synthesis[22]. In 2015, Liao et al. reported a substrate-controlled strategy for the enantioselective synthesis of two types of diarylindolylmethanes 4b-4c [Scheme 4][23]. Firstly, under chiral N-triflylphosphoramide CPA-3, N-methyl 2-substituted indoles were successfully employed as nucleophiles to achieve the 1,4-conjugate addition of aza-o-QM for the enantioselective synthesis of triarylmethanes 4b with indol-3-yl substitutions. Notably, an important enantioenriched spiroindoline 4k could be obtained by a NFSI-mediated cascade process of addition/deprotonation/deprotonation/spirocyclization from the compound 4f[24]. Subsequently, 3-substituted indoles were utilized as nucleophiles in this process to provide a series of chiral triarylmethanes 4c with indol-2-yl substitutions by optimizing the catalyst CPA-4. The author also demonstrated that the steric and electronic nature of the substituents had little influence on the reaction outcome, and all products could be delivered in high yields and good to excellent enantioselectivities.
Scheme 4. Asymmetric addition of aza-o-QMs with N-methyl 2-substituted indoles and 3-substituted indoles.
In 2016, Wang et al. developed an efficient enantioselective 1,4-addition of in situ generated o-QMs with electron-rich 2-naphthols [Scheme 5][25]. Different from Schneider’s work, diaryltosylmethane derivatives 5a were chosen as the precursor of o-QMs, and cinchonidine-derived squaramide CSA-1 was employed as organocatalyst. As a result, a range of the chiral triarylmethane derivatives 5b were obtained in good to high yields (up to 97% yield) with high enantioselectivities (up to 97% ee) under mild conditions. For mechanism, both the methoxy group and carbonyl group of the o-QM fragment were important H-bonding acceptors that can form hydrogen bonds with squaramide part of the catalyst CSA-1 at the same time. The proposed transition state 5-TS-I is the key for the stereo-controlled addition with 1-naphthols.
p-QMs. In their continuous work, Sun et al. designed and synthesized the tertiary alcohol derivatives 6a, which could act as the precursor of p-QMs [Scheme 6][26]. Then, starting from tertiary alcohols 6a, a mild catalytic asymmetric 1,6-conjugate addition was achieved to construct chiral triarylmethanes bearing all-carbon quaternary stereocenters. In this reaction, 2-substituted pyrroles were first utilized as electron-rich arene nucleophiles to give a range of chiral triarylmethanes 6c bearing pyrrol-2-yl groups with excellent efficiency and enantioselectivity. Through the screening of CPAs, indole can be compatible in this process. However, the author only gave one example, and ee value of the final product was 90%. They also confirmed that the 4-vinylphenol derivatives 6b were also competent substrates that could also generate p-QMs under the optimized conditions achieving an equally high enantioselective 1,6-addition. For mechanism, CPA-5 activated both pyrroles and p-QMs by forming two hydrogen bonds, and the larger δ substituent is oriented towards the back to minimize steric repulsion with the top-front bulky group, which was responsible for the stereo-control.
Scheme 6. Asymmetric addition of tertiary alcohols 6a or 4-vinylphenols 6b with 2-substituted pyrroles.
In their following work, 2-naphthols were also successfully employed in the asymmetric 1,6-addition of in situ generated p-QMs[27]. And this reaction was also catalyzed by chiral phosphoric acid CPA-5 and delivered a range of useful chiral triarylmethanes 7b containing a tertiary chiral stereocenters with good efficiency and enantioselectivity [Scheme 7].
Indeed, aryl boronic acids can also be used as aryl sources for the organocatalytic addition reaction[28]. Based on this, Huang et al. reported a chiral binaphthol (BINOL) catalyzed 1,6-addition of arylboronic acids to the presynthesized p-QMs 8a [Scheme 8][29]. The final chiral triarylmethanes 8b could be obtained in 61%-99% yields with excellent enantioselectivities. Mechanistically, the remote stereo-control of this reaction could be realized with the ortho-hydroxyl group which is designed on the δ-aryl group. Thus, both p-QMs and BINOL-1 can undergo dehydration with aryl boronic acids to form aryl borate intermediate 8-I which transfer into the products via an asymmetric intramolecular 1,6-addition. In addition, this designed ortho-hydroxyl group could also be utilized as a nucleophilic group in the following oxidation process to provide a spiro compound 8j.
In 2019, Wang et al. established a catalytic asymmetric conjugate addition of indoles to presynthesized p-QMs 9a in the presence of chiral phosphoric acid CPA-4, which afforded a range of chiral triarylmethanes 9b with indol-3-yl groups in generally high yields (54%-98%) and good enantioselectivities [Scheme 9][30]. After mechanism experiments, a more likely reaction path A was proposed. p-QMs 9a was transformed into o-QMs 9-I in the presence of CPA-4 first. Then, CPA-4 worked as a bifunctional organocatalyst to activate both o-QMs and indoles by forming two hydrogen bonds with the N-H group of indoles and the carbonyl group of o-QMs 9-I, thus facilitating an asymmetric 1,4-addition. Another proposed mechanism (path B) of CPA-4 catalyzed direct 1,6-addition is less likely, but it should not be totally excluded. Following the same strategy, Cheng et al. achieved a similar enantioselective conjugate addition of 2-naphthols to the presynthesized p-QMs 9a providing a series of enantioenriched triarylmethanes with 2-hydroxynaphthalen-1-yl groups[31].
Recently, a direct and enantioselective oxidative cross dehydrogenative coupling of racemic
Scheme 10. Enantioselective oxidative cross dehydrogenative coupling of 2,2-diarylacetonitriles 10a and electron-rich arenes.
Except for the above addition of p-QMs, the asymmetric reduction of p-QMs with δ,δ-diaryl substitutions was also an alternative strategy for chiral triarylmethanes synthesis. But the greatest difficulty of this strategy is the stereo-identification of two similar aryl groups on the δ position of p-QMs. In 2019, Fan and co-workers reported a Cu-catalyzed asymmetric reduction of the presynthesized p-QMs for the synthesis of chiral triarylmethanes[33], they designed an ortho-chlorine directing group on one of the two similar aryl groups. Owing to the crucial Cu-Cl interaction, an excellent asymmetric control has been achieved. More recently, Han et al. developed a chiral phosphoric acid CPA-7 catalyzed transfer hydrogenation of in situ generated p-QMs with benzothiazoline as the hydride source [Scheme 11][34]. A series of racemic triarylmethanols 11a participated in this reduction reaction smoothly, providing the corresponding enantioenriched triarylmethanes 11b in excellent yields and enantioselectivities. Compared with Fan’s work[33], this hydrogenation process took place under oraganocatalytic conditions and pre-synthesis of the p-QMs bearing bulky groups was unnecessary. Mechanism experiments revealed that the hydrogen bond donating ability of this directing group on the ortho-position was crucial for excellent enantiocontrol.
CONSTRUCTION OF CHIRAL TRIARYLMETHANES WITH INDOLE IMINE METHIDES
Similar to QMs, indole imine methides (IIMs) are another important family of active electrophiles facilitated by aromatization to indoles. Typically, IIMs are in situ generated by acid- or base-promoted elimination of a leaving group at the benzylic group of the corresponding indolyl compounds[35]. Since the pioneering work of Rueping’s group involving the in situ generation of the 3-indole imine methides (3-IIM) iminium ion pair[36], IIMs derivatives have become important electrophiles that can undergo nucleophilic attacking to give diverse compounds in synthetic chemistry[37-39]. If electron-rich arenes are selected as nucleophilic reagents, IIMs can undergo Friedel-Crafts type reaction to provide another efficient strategy for synthesizing triarylmethanes containing indolyl groups. It is worth mentioning that the nitrogen atom of IIMs can form a hydrogen bond with the organocatalysts to promote the stereo-control. So far, only 2-, 3-, 6- and 7-IIMs could be employed for the construction of chiral triarylmethanes 12 [Scheme 12].
3-IIMs. In 2009, Sun et al. developed a CPA-catalyzed tandem double Friedel-Crafts reaction of
Later on, Sun et al. reported a chiral phosphoric acid catalyzed Friedel-Crafts alkylation of electron-rich arenes with indol-3-yl methanamine 14a for the enantioselective synthesis of chiral triarylmethane 14b
Scheme 14. Asymmetric Friedel-Crafts alkylation of electron-rich arenes with (3-indolyl) methanamines.
In an attempt to improve the enatioselectivity of the Friedel-Crafts reaction of 3-IIMs, Zhuo et al. developed a chiral imidodiphosphoric acid CPA-10 catalyzed highly efficient and enantioselective Friedel-Crafts alkylations of TMS-protected 3-arylindolylmethanol 15a for the construction of chiral triarylmethanes
In their following research, Zhuo et al. developed a general method for the direct transformation of 3-hydroxy-3-indolyloxindoles 16a into 3,3-diaryloxindoles 16b-16c bearing triarylmethanes motif based on the activating strategy above [Scheme 16][43]. As a result, a range of quaternary carbon centered 3,3-diaryloxindoles 16b and 16c were synthesized in 75%-99% yield and 83%-98% ee with low catalyst loadings (as low as 0.5 mol%). Mechanistically, a similar 3-IIMs iminium ion pair intermediate 16-I generated from 16a and CPAs via dehydration was proposed to be the key intermediate for this transformation.
Scheme 16. Enantioselective Friedel-Crafts alkylations of 3-hydroxy-3-indolyloxindole 16a, HP-β-CD = hydroxypropyl-β-cyclodextrin.
As a further development, Zhang et al. utilized 3-vinyl indole 17a as the precursor of 3-IIMs to achieve a Friedel-Crafts reaction with indoles to afford the chiral triarylmethane 17b with all-carbon quaternary stereocenter [Scheme 17][44]. This method was a highly efficient atom-economic process and could provide the chiral triarylmethane 17b in excellent yields with excellent enantioselectivities. For mechanism, the acidic proton of CPA-13 protonated the 3-vinylindole 17a and formed a hydrogen bond with the stable 3-IIMs iminium intermediate 17-I. In addition, the basic P=O group of CPA-13 activated the indoles through the second hydrogen bond. The simultaneous formation of the two hydrogen bonds fixed 3-IIMs and indoles into a specific chiral pocket of CPA-13, which was the key factor in obtaining good enantioselectivities.
2-IIMs. In 2014, Qi et al. was the first to demonstrate the formation of 2-IIMs iminium intermediate from indol-2-yl methanols 18a through the CPA-catalyzed dehydration [Scheme 18][45]. 2-IIMs iminium intermediate 18-I then undergo an asymmetric 1,6-conjugate addition under a chiral environment with indoles to form highly enantioenriched 2,3’-diindolylarylmethane 18b. As a result, a series of
Converting nucleophiles from common indoles to 3-substituted indoles, Gong et al. developed a CPA‐catalyzed Friedel-Crafts reaction of indol-2-yl methanol 18a for the asymmetric contribution of triarylmethanes [Scheme 19][49]. This protocol provides an efficient method for constructing biologically important 2,2'‐bisindolylarylmethane 19b in high yields with moderate to good enantioselectivities. For mechanism, the author proposed that carbocation intermediate 19-I was initially generated from
Scheme 19. Enantioselective Friedel-Crafts alkylations of indol-2-yl methanols 18a and 3-substituted indoles.
In their subsequent work, Lan et al. employed 2-naphthols as nucleophiles in the strategy above, developing a convenient approach to the synthesis of chiral triarylmethanes [Scheme 20][50]. A series of diversified
Similar to Zhang et al.’s work, Han et al. utilized 2-vinyl indole derivative 21a as the precursor of 2-IIMs to achieve an enantioselective hydroarylation to give enantioenriched triarylmethanes bearing all-carbon quaternary stereocenter [Scheme 21][44,51]. As a result, a series of 2,3'-bis(indolyl)methanes 21b could be obtained with good functional group tolerance and excellent enantioselectivies. Mechanistically, this reaction begins with the protonation of the electron-rich C=C bond in the substrate by CPA-15 to generate the corresponding indolyl carbocation 21-I, which is indeed stabilized in an IIM form. Due to the different substituents on the methide carbon, this intermediate might be a mixture of Z/E isomers, which are in equilibrium with each other. The CPA then serves as a bifunctional catalyst to activate both the imine motif of the IIM intermediate and the indole nucleophile through two hydrogen bonds. The hydrogen bond between the catalyst and the imine motif prefers to place the small methyl group toward the chiral pocket (21-TS-II), because the large phenyl group in 21-TS-I experiences increased steric repulsion with the chiral catalyst. In the favored transition state 21-TS-I, the nucleophile is directed to the electrophilic site from the front bottom side by the hydrogen bond with the P=O group to form the final (R)-products. In addition, the final 2,3′-BIM product 21b generated in this reaction showed promising anticancer activities through preliminary biological activity tests.
In fact, nucleophiles can occur attacking on both C3 and C3' positions of 2-IIMs to provide the final products 22a and 22b [Scheme 22]. As described above, the nucleophilic attack of electron-rich arenes on C3' position of 2-IIMs provided an efficient strategy for the synthesis of chiral triarylmethanes. As we all know, to achieve nucleophilic attack on C3 position instead of C3' position, two bulky aryl groups substituted at C3' carbon of 2-IIMs were usually needed to help generate the intermediate 22-I which then undergo further aromatization or cycloisomerization to give final products 22b[52-56]. However, both two bulky aryl groups (R1 = R2) were almost the same, probably due to synthetic reasons.
Very recently, in order to verify what changes that might cause the differences between the two bulky aryl groups (R1 ≠ R2), Han et al. designed and synthesized a 2-indolylmethanol derivative 23a which has two different aryl groups on C3' position [Scheme 23][57]. Interestingly, they confirmed that a similar intermediate 22-I (R1 ≠ R2) could be generated after nucleophilic addition, which further undergoes aromatization involving a central-to-central chirality transfer progress to construct the chiral triarylmethanes. As the scope testing, a broad scope of indol-2-yl methanols 23a can be coupled with various indoles to produce chiral triarylmethane 23b in high yields with up to 96% ee value. The challenge in this work was the stereo identification of two aryl groups (Ar1vs Ar3). The author cleverly designed the methoxyl group on one of two aryl groups, and they confirmed that the hydrogen bonding between N-H and methoxyl group was the most important for distinguishing these two similar aryl groups. For the proposed mechanism, 2-indolylmethanol 23a could undergo dehydration to give the ion pair 23-I which could resonate with the CPA-activated indole imine methide 23-II. Although 2-IIM 23-II has not been successfully synthesized, the author believes that such a IIM 23-II might be a Z isomer due to the hydrogen bonding interaction between N-H and methoxyl group which is important for enantio-determination. Next, a CPA controlled enatioselective nucleophilic addition on C3 position of 23-II occurred to form chiral enamine specie 23-III. The next olefin isomerization to form final product 23b is thermodynamically driven by rearomatization. While concerted[1,3]-sigmatropic H-migration is forbidden, the author believes that this step can proceed with good enantioselective control by using CPA-16 as the proton shuttle via transition state 23-IV.
Fully substituted 2-IIMs (Scheme 22, R1 ≠ R2 ≠ H) can also undergo nucleophilic addition with hydrogen anion that provides a potential pathway for chiral triarylmethanes if R1 and R2 are both aryl groups. However, the biggest challenge of this approach is how to effectively distinguish the two similar aryl groups. In their previous work, Han et al. demonstrated that the methoxy group can be introduced as a directing group to identify these two similar aryls by the hydrogen bond between the methoxy group and the catalyst[34]. Very recently, Yan et al. also addressed this challenge by using a robust organocatalytic system leading to excellent enantioselection between aryl and heteroaryl groups without directing group in which versatile 2-indole imine methide 24-II bearing aryl and heteroaryl groups was employed [Scheme 24][58]. This protocol provided efficient access to a wide range of highly enantioenriched triarylmethane 24b bearing both indolyl and thienyl groups in good yields and excellent enantioselectivities from the corresponding racemic tertiary alcohol 24a. For mechanism, they proposed that an indolyl cation 24-I was first generated by pairing with a phosphate counter anion. This ion pair might be in equilibrium with the activated 2-IIMs 24-II. Subsequently, the hydride source approaches benzylic carbon to furnish the product 24b. Computational studies showed that the key interaction for the discrimination of these two aryl groups is mainly - stacking. Therefore, 24-TS-R was the major transition state because the electron-deficient thienyl is in closer contact with the electron-rich benzo ring of benzothiazoline. They also did a biological study that demonstrated the great potential of these triarylmethane 24b for anticancer and antiviral drug development.
6-IIMs and 7-IIMs. Compared with 3-IIMs and 2-IIMs, there is less research on other IIM derivatives, which may be difficult to synthesize their precursors. So far, only 6-IIMs and 7-IIMs have been successfully applied to contribute to the chiral triarylmethanes by asymmetric Friedel-Crafts reaction. In 2018, Wang et al. established the first catalytic asymmetric Friedel-Crafts reaction of indol-7-yl methanol 25a with tryptamine 25b as nucleophiles in the presence of CPA-18, which afforded a series of diverse chiral triarylmethane 25c with 7-indolyl group substitutions in moderate to good yields and generally excellent enantioselectivities [Scheme 25][59]. The mechanism study indicated that there was a kinetic resolution of 7-indolylmethanol 25a during the reaction process.
Mechanistically, they proposed two possible reaction pathways for this transformation [Scheme 26]. The active carbocation 26-I, which may exist stably in form 26-II, was first generated from indol-7-yl methanol 25a via dehydration under the catalysis of CPA-18. In the transition state, CPA-18 was proposed to activate 7-IIMs and tryptamine 25b simultaneously via hydrogen-bonding and ion-pairing interactions. Then, the transient intermediate 26-III was generated via nucleophilic trapping by the C2-position of tryptamine 25b. Finally, the corresponding triarylmethane 25c was formed after aromatization. Similar to pathway a, a dearomatized intermediate 26-TS-III was formed through the C3-attacking of tryptamine 25b. Then, intermediate C underwent a migration to generate a transient intermediate 26-TS-IV due to the driving force of rearomatization, which rapidly aromatized into final products.
Scheme 26. The proposed mechanism for asymmetric Friedel-Crafts reaction of indol-7-yl methanols 25a.
In the same year, Yue et al. employed 7-indolylmethanol 27a as the precursor of 7-IIMs to achieve enantioselective 1,4-arylations providing an efficient approach for the synthesis of diverse hetero-triarylmethanes 27b with indol-3-yl and indol-6-yl substitutions, which provided in high yields and excellent enantioselectivities [Scheme 27][60]. For mechanism, 7-IIMs were proposed to be the possible intermediate for this reaction. With control experiments investigation, they also suggested that a formal SN1 substitution was compatible with this catalytic system.
In their work, the author further extended the 1,4-addition reaction to the remote 1,8-addition reaction by utilizing the indol-6-yl methanols 28a as the precursor of 6-IIMs [Scheme 28]. As a result, a series of triarylmethanes 28b were provided in excellent yields and enantioselectivities. Mechanistically, the successful formation of 6-IIMs intermediate was suggested to be the key to the process.
CONSTRUCTION OF CHIRAL TRIARYLMETHANES WITH AZADIENES
Due to the driving force of aromatization, 2,3-dihydrobenzofuran fused azadienes, which can undergo nucleophilic 1,4-addition, have also been regarded as a pivotal class of highly reactive intermediates in organocatalytic asymmetric reactions for the synthesis of functional benzofuran derivatives[61-66]. If electron-rich arenes are selected as nucleophiles, the above 1,4-addition can also construct chiral triarylmethanes with 2-benzofuranyl substitution, which provides another alternative approach to chiral triarylmethanes constructions [Scheme 29].
In 2019, Zhang et al. developed an organocatalyst-controlled positionselectivity switchable enantioselective Friedel-Crafts reaction of 1-naphthols for the synthesis of two types of chiral triarylmethanes bearing naphthalen-2-yl groups [Scheme 30][67]. With cinchonidine-derived squaramide CSA-1 as organocatalyst, 1-naphthols underwent o-selective Friedel-Crafts alkylation to provide the first type of chiral triarylmethane 30b with 1-hydroxynaphthalen-2-yl group substitution. As a result, a series of azadiene 30a were employed in the Friedel-Crafts reaction to afford the corresponding products 30b in high yields (66%-99%) and enantioselectivities (71%-98%). This approach could tolerate both electron-withdrawing groups (including F, Cl, Br, etc.) and electron-donating groups (including Me, OMe, etc.).
Meanwhile, when chiral phosphoric acid CPA-20 was employed instead of squaramide CSA-1 as organocatalyst, a p-selective Friedel-Crafts alkylation of 1-naphthols could be achieved for the synthesis of second type chiral triarylmethanes with 1-hydroxynaphthalen-4-yl group substitution [Scheme 31]. The p-selective reaction also has good functional group tolerance to form a series of chiral triarylmethanes in 73%-99% yield with 70%-93% ee, which is the same as the o-selective Friedel-Crafts alkylation. Most importantly, no isomeric o-selective products were formed to a detectable extent under this reaction condition. Although the author did not propose a detailed mechanism, the results of control experiments indicated that the free hydroxyl group of 1-naphthols played a key role in this catalyst-controlled position-selectivity switchable enantioselective Friedel-Crafts alkylation, probably due to the hydrogen bonding formation.
Indoles could also be utilized as electronic-rich arenes in the Michael addition of azadienes for chiral triarylmethanes synthesis which was first revealed by Xie et al. With chiral phosphoric acid CPA-15 as organocatalyst, a broad scope of indoles underwent conjugate addition to azadienes preparing a series of structurally important indolyl and benzofuranyl based triarylmethanes 32a in excellent yields and enantioselectivities [Scheme 32][68]. The author proposed a mechanism that chiral phosphoric acid acted as a bifunctional catalyst to active the NH group of the indoles and the imine group of the azadienes by the hydrogen bonding, which facilitates the enantioselective 1,4-conjugate addition of indole to azadiene 30a to give the final products 32a.
Recently, by using chiral phosphoric acid CPA-5 as catalyst, Lu et al. also developed an efficient catalytic enantioselective 1,4-addition of azadienes with 3-methyl indoles which provided a promising approach for the enantioselective construction of structurally diverse 2-indolyl triarylmethane derivatives 33b with moderate to good yields (up to 98%) and moderate to excellent enantioselectivities(up to 99% ee)
In 2020, Wang et al. achieved an enantioselective 1,4-conjugate addition of 1-azadienes with 2-naphthols as the nucleophiles for triarylmethanes bearing 2-hydroxynaphthalen-1-yl group synthesis by applying cinchonidine-driven urea catalyst CUC-1 [Scheme 34][70]. This protocol efficiently enabled access to a variety of important benzofuran-containing hetero-triarylmethanes 34a in up to 98% yields and 99% ee. Electron-rich phenol derivatives could also be compatible in this catalytic system, such as the synthesis of product 34g. As shown in Scheme 34, both 2-naphthols and azadienes were activated by CUC-1 via hydrogen bonding to finish this reaction through the favored transition state 34-TS-I.
As a continuous work, Xie et al. recently developed 1,4-addition of azadienes with pyrazolin-5-ones providing a series of chiral triarylmethanes 35a bearing pyrazole moiety in moderate to excellent yields with excellent enantioselectivities [Scheme 35][71]. In this reaction, cinchonidine-drived squaramide CSA-2 exhibited the optimal catalytic activity, which acted as a bifunctional organocatalyst. In the proposed catalytic model 35-TS-I, the Brønsted base part of squaramide catalyst CSA-2 captured the proton of pyrazol-5-ol intermediate and the Brønsted acid part of squaramide activated the imine group of azadiene via hydrogen bonding, then the final products 35a were given by the following enantioselective Michael addition, and base-catalyzed tautomerization.
CONSTRUCTION OF CHIRAL TRIARYLMETHANES VIA OTHERS METHODS
The chiral 3,3’-diaryloxindoles with structural characteristics of triarylmethane unit are biologically interesting compounds[72-75]. In 2014, Shirakawa et al. reported a phase-transfer-catalyzed SNAr reaction of
As an alternative strategy, the desymmetrization of achiral triarylmethanes was also efficient in obtaining the enantioenriched triarylmethanes [Scheme 37], among which Pd-catalyzed desymmetric C-H arylation of achiral triarylmethanes developed by Yu group is the most popular strategy (not shown)[77-79].
Scheme 37. The strategy of chiral triarylmethanes forms a desymmetrization of achiral triarylmethanes.
Until 2017, Lu et al. developed an efficient process for chiral triarylmethanes synthesis through a
CHIRAL TETRAARYLMETHANES SYNTHESIS
Compared with triarylmethanes, the synthesis of tetraarylmethanes is more challenging, especially in the chiral version, which lies in not only the high barrier in making the extremely congested C-C bond connecting the central carbon and new aryl rings, but also the difficult stereo-identification between the existing similar aryl rings with the new introduced aryl ring. Therefore, there has been a lack of methods to directly construct the chiral tetraarylmethanes with concomitant establishment of the quaternary stereogenic center[81]. Until 2020, Li et al. extended the 1,6-addition strategy of p-QMs employing pyrroles as nucleophiles for the synthesis of chiral tetraarylmethanes, triarylmethanol 39a were introduced in the catalytic system, in which they skillfully designed two similar aryl groups (Ar2 and Ar3) at δ-positions of the in-situ generated p-QMs and these two similar aryl groups were identified by a “tag group”, the identification of these two similar aryl groups could be achieved by the hydrogen bonding interaction between the direction group and the CPA catalyst, thus compensating the shortage of direct construction of chiral tetraarylmethane 39b [Scheme 39][82]. Similarly, the triarylmethanol 39k as precursors of 2-IIMs were also expanded into the asymmetric synthesis of chiral tetraarylmethane 39l bearing indol-2-yl groups. As a result, a series of chiral tetraarylmethanes 39b and 39l were provided in good yields and enantioselectivities. Furthermore, the biological activity experiments indicate that these bright new chiral products are highly promising anticancer agents. For mechanism, the ortho-methoxy group considered as directing group was also replaced with o-Me, m-OMe, p-OMe, o-CH2OMe or o-Et, resulting in a uniformly dramatic decrease in enantioselectivity, suggesting that this o-OMe group mainly functions as a hydrogen-bond donor.
Combined with density functional theory calculations, they give a possible reaction process, as shown in Scheme 40. Firstly, the chiral phosphoric acid CPA-7 forms a hydrogen bond with the substrate 39a to generate the intermediate 39-I which then undergoes dehydration via 39-TS-I to afford an identical p-QM intermediate 39-II, requiring an activation free energy of 21.2 kcal/mol. Next, the nucleophile 2-methyl pyrrole attacks the p-QM intermediate via 39-TS-II releasing free energy of 15.8 kcal mol-1 to give the intermediate 39-III, which was considered to be the enantio-determining step. The subsequent intermolecular proton shift process via 39-TS-III gave the final products.
In their subsequent work, Li et al. developed a catalytic asymmetric formal cross dehydrogenative coupling process for the construction of chiral tetraarylmethanes, racemic triarylmethane 41a underwent oxidation to form a p-QMs intermediate, then, the asymmetric 1,6-addition of p-QMs occurred following the same catalytic process to finish the reaction [Scheme 41][83]. As a result, a series of enantioenriched tetraarylmethanes were prepared in both excellent yield and enantioselectivity. It is worth mentioning that these tetraarylmethane products 41b were verified to have promising antiviral activity.
Scheme 41. The contribution of chiral tetraarylmethanes through a formal cross dehydrogenative coupling.
It was well known that benzylic carbocations bearing an ortho- or para-hydroxyl group can be stabilized by forming o-QMs or p-QMs, which has been summarized above. However, those benzylic carbocations with meta-hydroxyl group have remained almost unexplored in organic synthesis due to the lacking of resonance stabilization by a typical quinone methide form. Very recently, Tan et al. reported a novel and catalytic enantioselective addition of meta-hydroxyl triarylmethanols 42a with indoles via triaryl carbocation intermediates, providing an efficient access to chiral tetraarylmethanes with excellent enantiocontrol
CONCLUSION AND OUTLOOK
This review has summarized a series of efficient methods for the synthesis of chiral triarylmethanes and chiral tetraarylmethanes with structural diversity and complexity under organocatalytic conditions. The whole field has only been developed for about 15 years since the pioneer report was published. Therefore, it is still in its infancy. For the development of this field, the following directions can be considered: (a) Although the types of electrophilic reagents have been extensively developed, the types of nucleophiles are still limited in electron-rich arenes, focussing on indoles, pyrroles and naphthols. Therefore, the expansion of electron-rich arenes is worth studying; (b) At present, the synthesis of chiral triarylmethanes mainly relies on the asymmetric Friedel-Crafts reaction and conjugate addition of electron-rich arenes. The development of new construction strategies and new catalytic models is also a hot topic in this field; (c) With more synthetic challenges, chiral tetraarylmethanes have shown great potential in anticancer activities due to their unique chiral spherical structures; however, there are few efficient strategies to achieve their synthesis. Therefore, more research can be focused on the synthesis of chiral tetraarylmethanes; (d) The development of synthetic methods needs to be further expanded to the synthesis of known active molecules and natural products, and the potential realization of chiral triarylmethanes in the field of drug development is also an encouraging research direction.
DECLARATIONS
Authors’ contributions
Prepared and corrected the manuscript: Han Z, Liu R, Huang H
Availability of data and materials
Not applicable.
Financial support and sponsorship
This work was supported by the NSFC (22101033, 22201023); the Jiangsu Key Laboratory of Advanced Catalytic Materials and Technology (BM2012110); the Natural Science Foundation of Jiangsu Province (BK20210849); and the Innovation & Entrepreneurship Talents Plan of Jiangsu Province (JSSCRC2021536).
Conflicts of interest
All authors declared that there are no conflicts of interest.
Ethical approval and consent to participate
Not applicable.
Consent for publication
Not applicable.
Copyright
© The Author(s) 2023.
REFERENCES
1. Duxbury DF. The photochemistry and photophysics of triphenylmethane dyes in solid and liquid media. Chem Rev 1993;93:381-433.
2. Shchepinov MS, Korshun VA. Recent applications of bifunctional trityl groups. Chem Soc Rev 2003;32:170-80.
3. Nair V, Thomas S, Mathew SC, Abhilash K. Recent advances in the chemistry of triaryl-and triheteroarylmethanes. Tetrahedron 2006;62:6731-47.
4. Mondal S, Panda G. Synthetic methodologies of achiral diarylmethanols, diaryl and triarylmethanes (TRAMs) and medicinal properties of diaryl and triarylmethanes-an overview. RSC Adv 2014;4:28317-58.
5. Nambo M, Crudden CM. Recent advances in the synthesis of triarylmethanes by transition metal catalysis. ACS Catal 2015;5:4734-42.
6. Saha S, Alamsetti SK, Schneider C. Chiral Brønsted acid-catalyzed Friedel-Crafts alkylation of electron-rich arenes with
7. Caruana L, Fochi M, Bernardi L. The Emergence of quinone methides in asymmetric organocatalysis. Molecules 2015;20:11733-64.
8. Dorsch C, Schneider C. Asymmetric Brønsted acid catalyzed cycloadditions of ortho-quinone methides and related compounds. Synthesis 2022;54:3125-41.
9. Groenendaal B, Ruijter E, Orru RV. 1-Azadienes in cycloaddition and multicomponent reactions towards N-heterocycles. Chem Commun 2008;43:5474-89.
10. Fell JS, Martin BN, Houk KN. Origins of the unfavorable activation and reaction energies of 1-azadiene heterocycles compared to
11. Mondal S, Roy D, Panda G. Overview on the recent strategies for the enantioselective synthesis of 1, 1-Diarylalkanes, triarylmethanes and related molecules containing the diarylmethine stereocenter. ChemCatChem 2018;10:1941-67.
12. Pathak TP, Sigman MS. Applications of ortho-quinone methide intermediates in catalysis and asymmetric synthesis. J Org Chem 2011;76:9210-5.
13. Bai WJ, David JG, Feng ZG, Weaver MG, Wu KL, Pettus TR. The domestication of ortho-quinone methides. Acc Chem Res 2014;47:3655-64.
14. Jaworski AA, Scheidt KA. Emerging roles of in situ generated quinone methides in metal-free catalysis. J Org Chem 2016;81:10145-53.
15. Wang Z, Sun J. Recent advances in catalytic asymmetric reactions of o-Quinone methides. Synthesis 2015;47:e7-e7.
16. Li W, Xu X, Zhang P, Li P. Recent advances in the catalytic enantioselective reactions of para-Quinone methides. Chem Asian J 2018;13:2350-9.
17. Lima CGS, Pauli FP, Costa DCS, et al. para-Quinone methides as acceptors in 1,6-Nucleophilic conjugate addition reactions for the synthesis of structurally diverse molecules. Eur J Org Chem 2020;2020:2650-92.
18. Kikuchi J, Terada M. Enantioconvergent substitution reactions of racemic electrophiles by organocatalysis. Chemistry 2021;27:10215-25.
19. Ma Y, He X, Yang Q, Boucherif A, Xuan J. Recent advances in organocatalytic asymmetric cycloaddition reactions through
20. Zhang RL, Liu B, Qiu KX, et al. Asymmetric Synthesis of Triphenylmethanes via Organocatalytic Regio- and enantioselective
21. Zhao W, Wang Z, Chu B, Sun J. Enantioselective formation of all-carbon quaternary stereocenters from indoles and tertiary alcohols bearing a directing group. Angew Chem Int Ed Engl 2015;54:1910-3.
23. Liao HH, Chatupheeraphat A, Hsiao CC, Atodiresei I, Rueping M. Asymmetric Brønsted acid catalyzed synthesis of
24. Nyffeler PT, Durón SG, Burkart MD, Vincent SP, Wong CH. Selectfluor: mechanistic insight and applications. Angew Chem Int Ed Engl 2004;44:192-212.
25. Wang Y, Zhang C, Wang H, Jiang Y, Du X, Xu D. Bifunctional amine-squaramide catalyzed friedel-crafts alkylation based on
26. Wang Z, Wong YF, Sun J. Catalytic asymmetric 1,6-Conjugate addition of para-Quinone methides: formation of all-carbon quaternary stereocenters. Angew Chem Int Ed Engl 2015;54:13711-4.
27. Wong YF, Wang Z, Sun J. Chiral phosphoric acid catalyzed asymmetric addition of naphthols to para-quinone methides. Org Biomol Chem 2016;14:5751-4.
28. Han WY, Wu ZJ, Zhang XM, Yuan WC. Enantioselective organocatalytic three-component Petasis reaction among salicylaldehydes, amines, and organoboronic acids. Org Lett 2012;14:976-9.
29. Huang G, Huang W, Guo J, et al. Enantioselective synthesis of triarylmethanes via organocatalytic 1,6-Addition of arylboronic acids to para-Quinone methides. Adv Synth Catal 2019;361:1241-6.
30. Wang JR, Jiang XL, Hang QQ, Zhang S, Mei GJ, Shi F. Catalytic asymmetric conjugate addition of indoles to para-quinone methide derivatives. J Org Chem 2019;84:7829-39.
31. Cheng Y, Fang Z, Jia Y, Lu Z, Li W, Li P. Organocatalytic enantioselective conjugate addition of 2-naphthols to ortho-hydroxyphenyl substituted para-quinone methides: access to unsymmetrical triarylmethanes. RSC Adv 2019;9:24212-7.
32. Wang Z, Zhu Y, Pan X, Wang G, Liu L. Synthesis of chiral triarylmethanes bearing all-carbon quaternary stereocenters: catalytic asymmetric oxidative cross-coupling of 2,2-Diarylacetonitriles and (Hetero)arenes. Angew Chem Int Ed Engl 2020;59:3053-7.
33. Pan T, Shi P, Chen B, et al. CuH-Catalyzed asymmetric 1,6-Conjugate reduction of p-Quinone methides: enantioselective synthesis of triarylmethanes and 1,1,2-triarylethanes. Org Lett 2019;21:6397-402.
34. Han Z, Zang Y, Liu C, Guo W, Huang H, Sun J. Enantioselective synthesis of triarylmethanes via organocatalytic transfer hydrogenation of para-quinone methides. Chem Commun 2022;58:7128-31.
35. Wang L, Chen Y, Xiao J. Alkylideneindoleninium ions and alkylideneindolenines: key intermediates for the asymmetric synthesis of 3-Indolyl derivatives. Asian J Org Chem 2014;3:1036-52.
36. Rueping M, Nachtsheim BJ, Moreth SA, Bolte M. Asymmetric Brønsted acid catalysis: enantioselective nucleophilic substitutions and 1,4-additions. Angew Chem Int Ed Engl 2008;47:593-6.
37. Dalpozzo R. Strategies for the asymmetric functionalization of indoles: an update. Chem Soc Rev 2015;44:742-78.
38. Mei GJ, Shi F. Indolylmethanols as reactants in catalytic asymmetric reactions. J Org Chem 2017;82:7695-707.
39. Petrini M. New Perspectives in the indole ring functionalization using 2-Indolylmethanols. Adv Synth Catal 2020;362:1214-32.
40. Sun FL, Zeng M, Gu Q, You SL. Enantioselective synthesis of fluorene derivatives by chiral phosphoric acid catalyzed tandem double Friedel-Crafts reaction. Chemistry 2009;15:8709-12.
41. Sun F, Zheng X, Gu Q, He Q, You S. Enantioselective synthesis of unsymmetrical triarylmethanes by chiral Brønsted Acids. Eur J Org Chem 2010;2010:47-50.
42. Zhuo MH, Jiang YJ, Fan YS, Gao Y, Liu S, Zhang S. Enantioselective synthesis of triarylmethanes by chiral imidodiphosphoric acids catalyzed Friedel-Crafts reactions. Org Lett 2014;16:1096-9.
43. Zhuo M, Liu G, Song S, et al. Chiral imidodiphosphoric acids-catalyzed friedel-crafts reactions of indoles/pyrroles with
44. Zhang Y, Zhang S, Fu L, Guo Q. Highly Efficient Atom-Economic Synthesis of Chiral Bis(indolyl)methanes Bearing Quaternary Stereogenic Carbon Centers. ChemCatChem 2017;9:3107-10.
45. Qi S, Liu CY, Ding JY, Han FS. Chiral phosphoramide-catalyzed enantioselective synthesis of 2,3'-diindolylarylmethanes from
46. Qi S, Kang CQ, Han FS. The determination of the absolute configuration of a chiral 2,3'-diindolylarylmethane by NMR spectroscopy. Magn Reson Chem 2015;53:181-7.
47. Li X, Li Z, Sun J. Quinone methides and indole imine methides as intermediates in enantioselective catalysis. Nat Synth 2022;1:426-38.
48. Zhang YC, Jiang F, Shi F. Organocatalytic asymmetric synthesis of indole-based chiral heterocycles: strategies, reactions, and outreach. Acc Chem Res 2020;53:425-46.
49. Gong YX, Wu Q, Zhang HH, Zhu QN, Shi F. Enantioselective construction of a 2,2'-bisindolylmethane scaffold via catalytic asymmetric reactions of 2-indolylmethanols with 3-alkylindoles. Org Biomol Chem 2015;13:7993-8000.
50. Lan J, Lu Y, Chen K, et al. Catalytic asymmetric substitution reaction of 3-Substituted 2-Indolylmethanols with 2-Naphthols. Synthesis 2020;52:3684-92.
51. Han Z, Wang W, Zhuang H, et al. Catalytic enantioselective synthesis of 2,3'-Bis(indolyl)methanes bearing all-carbon quaternary stereocenters via 2-Indole imine methides. Org Lett 2023;25:477-82.
52. Sun XX, Zhang HH, Li GH, He YY, Shi F. Catalytic enantioselective and regioselective [3 + 3] cycloadditions using
53. Zhu Z, Shen Y, Sun X, Tao J, Liu J, Shi F. Catalytic asymmetric [3 + 2] cycloadditions of C-3 unsubstituted 2-indolylmethanols: regio-, diastereo- and enantioselective construction of the cyclopenta[b]indole framework. Adv Synth Catal 2016;358:3797-808.
54. Li C, Zhang HH, Fan T, Shen Y, Wu Q, Shi F. Brønsted acid-catalyzed regioselective reactions of 2-indolylmethanols with cyclic enaminone and anhydride leading to C3-functionalized indole derivatives. Org Biomol Chem 2016;14:6932-6.
55. Zhang HH, Wang CS, Li C, Mei GJ, Li Y, Shi F. Design and enantioselective construction of axially chiral naphthyl-indole skeletons. Angew Chem Int Ed Engl 2017;56:116-21.
56. Zhu ZQ, Shen Y, Liu JX, Tao JY, Shi F. Enantioselective direct α-arylation of pyrazol-5-ones with 2-indolylmethanols via
57. Han Z, Zhuang H, Tang L, et al. Catalytic asymmetric allylic substitution/isomerization with central chirality transposition. Org Lett 2022;24:4246-51.
58. Yan Q, Duan M, Chen C, et al. Organocatalytic discrimination of non-directing aryl and heteroaryl groups: enantioselective synthesis of bioactive indole-containing triarylmethanes. Chem Sci 2022;13:5767-73.
59. Wang H, Xu M, Wan Y, Mao Y, Mei G, Shi F. Application of 7-Indolylmethanols in catalytic asymmetric arylations with tryptamines: enantioselective synthesis of 7-indolylmethanes. Adv Synth Catal 2018;360:1850-60.
60. Yue C, Na F, Fang X, Cao Y, Antilla JC. Chiral phosphoric acid catalyzed asymmetric synthesis of hetero-triarylmethanes from racemic indolyl alcohols. Angew Chem Int Ed Engl 2018;57:11004-8.
61. Ni H, Tang X, Zheng W, Yao W, Ullah N, Lu Y. Enantioselective phosphine-catalyzed formal [4 + 4] annulation of α,β-unsaturated imines and allene ketones: construction of eight-membered rings. Angew Chem Int Ed Engl 2017;56:14222-6.
62. Gu Z, Zhou J, Jiang G, Zhou Y. Synthesis of chiral γ-aminophosphonates through the organocatalytic hydrophosphonylation of azadienes with phosphites. Org Chem Front 2018;5:1148-51.
63. Gu Z, Xie J, Jiang G, Zhou Y. Catalytic asymmetric conjugate addition of tritylthiol to azadienes with a bifunctional organocatalyst. sian J Org Chem 2018;7:1561-4.
64. Gu Z, Wu B, Jiang G, Zhou Y. Synthesis of benzofuran-fused 1,4-Dihydropyridines via bifunctional squaramide-catalyzed formal
65. Gao ZH, Chen KQ, Zhang Y, Kong LM, Li Y, Ye S. Enantioselective N-Heterocyclic carbene-catalyzed synthesis of spirocyclic oxindole-benzofuroazepinones. J Org Chem 2018;83:15225-35.
66. Wang CS, Li TZ, Cheng YC, Zhou J, Mei GJ, Shi F. Catalytic asymmetric [4 + 1] cyclization of benzofuran-derived azadienes with
67. Zhang C, Cheng Y, Li F, Luan Y, Li P, Li W. organocatalytic enantioselective regiodivergent c-h bond functionalization of
68. Xie H, Wu B, Wang X, Zhou Y. Chiral Brønsted acid-catalyzed conjugate addition of indoles to azadienes: Enantioselective synthesis of hetero-triarylmethanes. Chin J Catal 2019;40:1566-75.
69. Lu Z, Hu J, Lan W, et al. Enantioselective synthesis of hetero-triarylmethanes by chiral phosphoric acid-catalyzed 1,4-addition of
70. Wang C, Yang Q, Wang M, et al. Catalytic asymmetric 1,4-type Friedel-Crafts (hetero)arylations of 1-azadienes: the highly enantioselective syntheses of chiral hetero-triarylmethanes. Org Chem Front 2020;7:609-16.
71. Xie H, Wu B, Chen M, et al. Enantioselective synthesis of triarylmethanes bearing pyrazole moiety through squaramide-catalyzed addition of azadienes with pyrazolin-5-ones. Chin J Org Chem 2020;40:3452.
72. Natarajan A, Guo Y, Harbinski F, et al. Novel arylsulfoanilide-oxindole hybrid as an anticancer agent that inhibits translation initiation. J Med Chem 2004;47:4979-82.
73. Neel DA, Brown ML, Lander PA, et al. 3,3-Bisaryloxindoles as mineralocorticoid receptor antagonists. Bioorg Med Chem Lett 2005;15:2553-7.
74. Uddin MK, Reignier SG, Coulter T, et al. Syntheses and antiproliferative evaluation of oxyphenisatin derivatives. Bioorg Med Chem Lett 2007;17:2854-7.
75. Christensen MK, Erichsen KD, Trojel-Hansen C, et al. Synthesis and antitumor effect in vitro and in vivo of substituted 1,
76. Shirakawa S, Koga K, Tokuda T, Yamamoto K, Maruoka K. Catalytic asymmetric synthesis of 3,3'-diaryloxindoles as triarylmethanes with a chiral all-carbon quaternary center: phase-transfer-catalyzed S(N)Ar reaction. Angew Chem Int Ed Engl 2014;53:6220-3.
77. Shi BF, Maugel N, Zhang YH, Yu JQ. Pd(II)-catalyzed enantioselective activation of C(sp2)-H and C(sp3)-H bonds using monoprotected amino acids as chiral ligands. Angew Chem Int Ed Engl 2008;47:4882-6.
78. Chu L, Wang XC, Moore CE, Rheingold AL, Yu JQ. Pd-catalyzed enantioselective C-H iodination: asymmetric synthesis of chiral diarylmethylamines. J Am Chem Soc 2013;135:16344-7.
79. Laforteza BN, Chan KS, Yu JQ. Enantioselective ortho-C-H cross-coupling of diarylmethylamines with organoborons. Angew Chem Int Ed Engl 2015;54:11143-6.
80. Lu S, Song X, Poh SB, Yang H, Wong MW, Zhao Y. Access to enantiopure triarylmethanes and 1,1-diarylalkanes by nhc-catalyzed acylative desymmetrization. Chemistry 2017;23:2275-81.
81. Tsuchida K, Senda Y, Nakajima K, Nishibayashi Y. Construction of chiral Tri- and tetra-arylmethanes bearing quaternary carbon centers: copper-catalyzed enantioselective propargylation of indoles with propargylic esters. Angew Chem Int Ed Engl 2016;55:9728-32.
82. Li X, Duan M, Deng Z, et al. Catalytic enantioselective synthesis of chiral tetraarylmethanes. Nat Catal 2020;3:1010-9.
83. Li Z, Li Y, Li X, Wu M, He ML, Sun J. Organocatalytic asymmetric formal oxidative coupling for the construction of all-aryl quaternary stereocenters. Chem Sci 2021;12:11793-8.
Cite This Article
Export citation file: BibTeX | RIS
OAE Style
Han Z, Liu R, Huang H. The recent advances in the contribution of chiral triarylmethanes and tetraarylmethanes with organocatalysts. Chem Synth 2023;3:32. http://dx.doi.org/10.20517/cs.2023.11
AMA Style
Han Z, Liu R, Huang H. The recent advances in the contribution of chiral triarylmethanes and tetraarylmethanes with organocatalysts. Chemical Synthesis. 2023; 3(4): 32. http://dx.doi.org/10.20517/cs.2023.11
Chicago/Turabian Style
Han, Zhengyu, Ruizhi Liu, Hai Huang. 2023. "The recent advances in the contribution of chiral triarylmethanes and tetraarylmethanes with organocatalysts" Chemical Synthesis. 3, no.4: 32. http://dx.doi.org/10.20517/cs.2023.11
ACS Style
Han, Z.; Liu R.; Huang H. The recent advances in the contribution of chiral triarylmethanes and tetraarylmethanes with organocatalysts. Chem. Synth. 2023, 3, 32. http://dx.doi.org/10.20517/cs.2023.11
About This Article
Special Issue
Copyright
Author Biographies
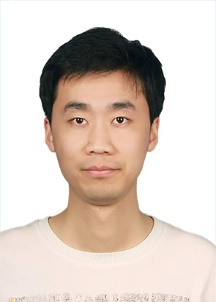
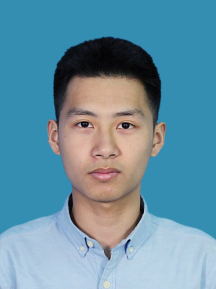
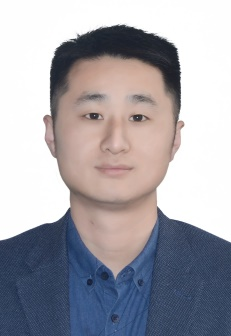
Data & Comments
Data

Comments
Comments must be written in English. Spam, offensive content, impersonation, and private information will not be permitted. If any comment is reported and identified as inappropriate content by OAE staff, the comment will be removed without notice. If you have any queries or need any help, please contact us at support@oaepublish.com.